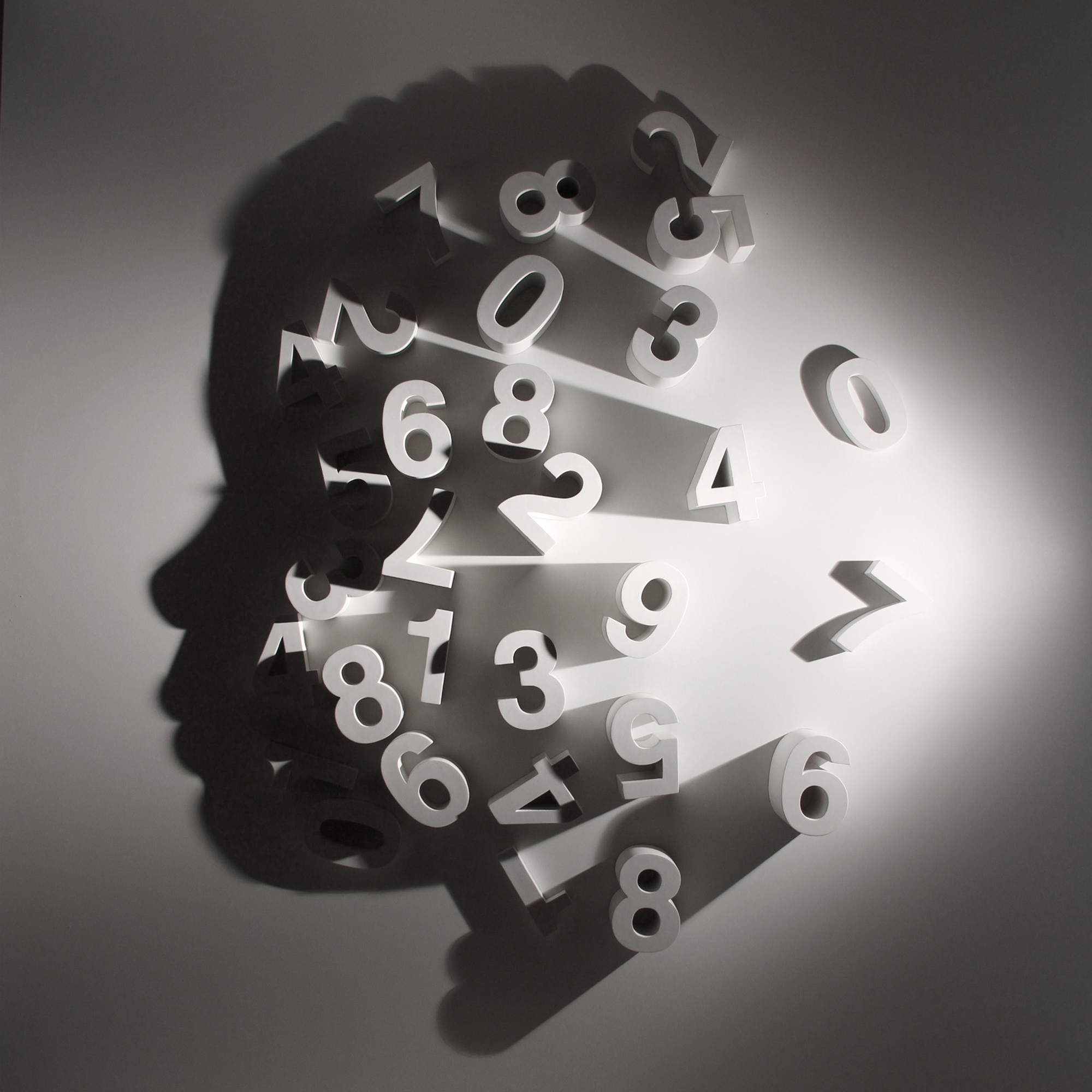
Feature
Realizing the potential of Neuroscience and Technology to transform Education
February 09, 2021
Just as the burgeoning understanding of biology in the nineteenth century transformed medicine, our new understanding of how the brain learns is set to transform education in the twenty-first century.
©Kumi Yamashita
These include misconceptions about the brain—so-called “neuromyths”. However, as was the case with medicine, science is beginning to shine a light into the darkness, and help provide a progressive, research-informed basis for the decisions we make in education policy and practice.
By Paul Howard-Jones, Ross Cunnington, Vivian Reigosa-Crespo, Juan Valle Lisboa,
09/02/ 2021
·
- Share
Looking inside the living, learning brain
Mutually supportive developments in technology and neuroscience research are producing rapid advances in our understanding of the learning brain. Until the 1990s, most of what we understood about the brain was derived from animal studies and the effects of injury on humans. For example, in the nineteenth century, the French physician and anthropologist Paul Broca discovered a speech production centre in the left frontal region of the brain (now called Broca’s area) after observing that a patient with damage in this region had difficulty in articulating speech, despite being able to understand language normally.
Discoveries such as Broca’s played a significant role in the development of neuroscience. However, it is obvious that the observational methods used were unable to map the complex brain processes by which healthy adults and children learn, or to enhance our understanding of how different interventions can enhance this learning. The development of in vivo neuroimaging made the non-invasive observation of the living human brain possible. This has dramatically accelerated scientific progress in understanding how the brain functions—including how we learn. Using technologies such as functional Magnetic Resonance Imaging (fMRI), we can now show how different regions of the brain activate and connect when we learn. This has allowed scientists to piece together the mechanisms involved, and discover how different factors impact these mechanisms.
he following four cases—all examples of real impact arising from the application of neuroscientific research—highlight some of the ways in which these neuroscience technologies are transforming our understanding of how the brain learns.
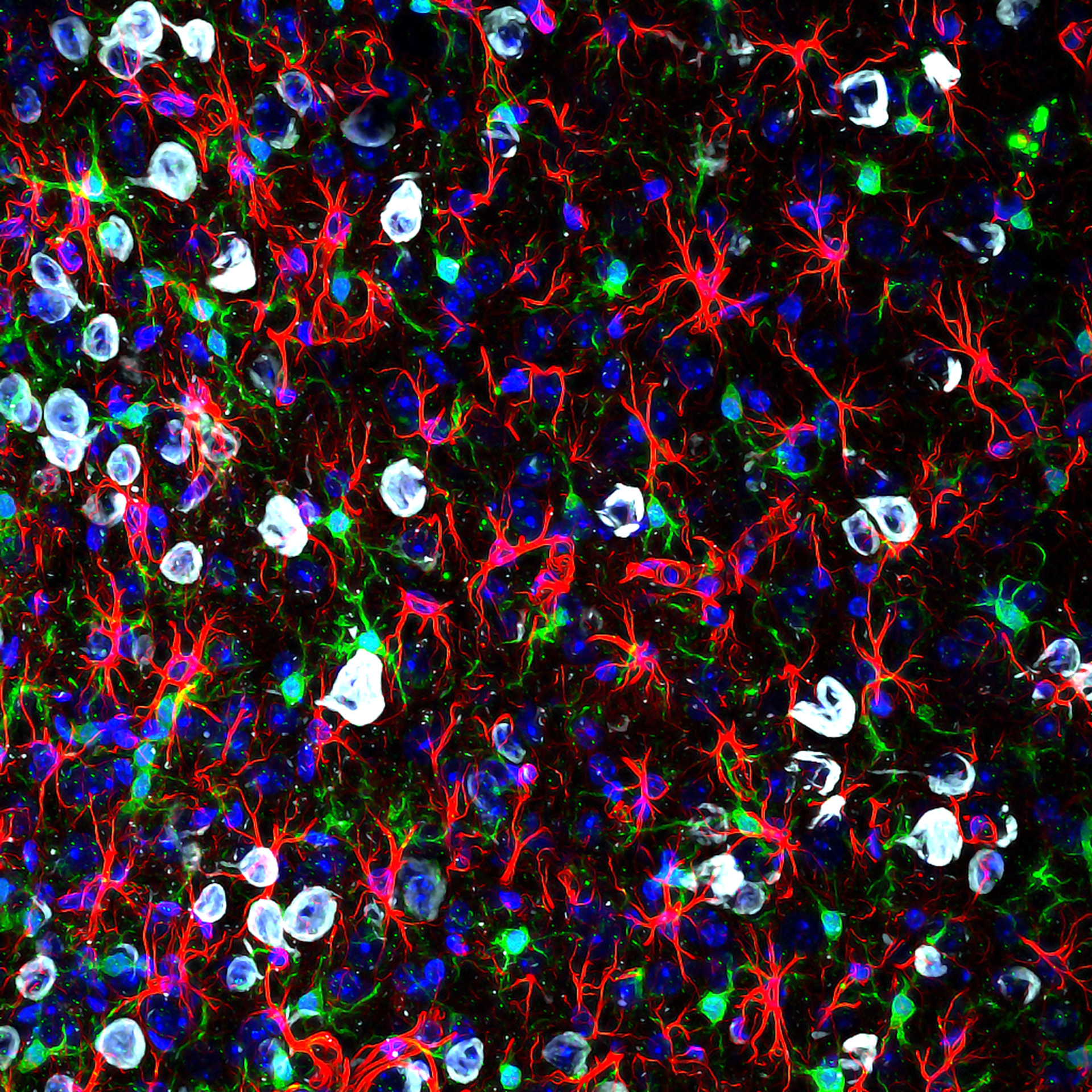
Selected region of the hippocampus of a mouse brain, showing tau inclusions (in white)—a shared feature of many neurodegenerative diseases. ©Gabe Luna
Using functional magnetic resonance imaging (fMRI) to understand how we learn
With fMRI, researchers can measure brain activity by detecting changes associated with blood flow. It involves an individual lying in a very strong magnetic field (about 10,000 times the strength of the Earth’s magnetic field), which is then pulsed. The blood flow can be calculated based on how the field responds. This technology of fMRI allows scientists to detect which parts of the brain are activating or deactivating in response to learning, since learning (or any type of mental activity) involves changes in activity in different brain regions, with consequent changes in blood flow in those regions.
In one recent study in the UK, researchers looked at how “gamifying” learning can improve the rate at which students learn. Adult participants were asked to study a range of topics, from ancient history to mathematics. They experienced the learning through three modes: reading notes, as a self-test quiz game, and as a competitive quiz game involving escalating rewards won on a wheel of fortune. They were tested on their knowledge before and after their study session inside the brain scanner, and again a few weeks later. Researchers found that the more the learning was presented as a game, the more the rate of learning increased. At the same time, increased gamification also meant that less activity was observed in the brain network associated with mind-wandering, the so-called “default mode network”. In fact, students’ marks in a consequent test could be predicted by how much this mind-wandering network was inhibited. The game’s advantage for learning was that it kept students alert and focused on the external world in front of them, discouraging them from becoming lost in unhelpful reverie while studying. Activation of the default mode network may also be a helpful “marker” of engagement in future educational research of this type.
The growth in our understanding of how learning occurs in the classroom has immense potential value for educators. Teachers continuously make decisions that impact on their students’ learning, and it is simply not possible to furnish them with appropriate instructions for what they should do in every situation. Indeed, the extent to which a teacher simply applies prescribed good practice has been found to be a poor predictor of effective learning. Instead, teachers must be able to constantly select and adapt approaches based on their own understanding of classroom learning. A scientific grasp of how learning happens can help here, supporting teachers in getting more out of their students. Gamification represents one very useful tool in doing this, and understanding how it works can help teachers gamify their lessons more effectively. Not surprisingly, teachers are now encountering learning concepts from mind and brain science in their initial teacher training and continuing professional development. There is more to be done, of course, but this constitutes important progress.
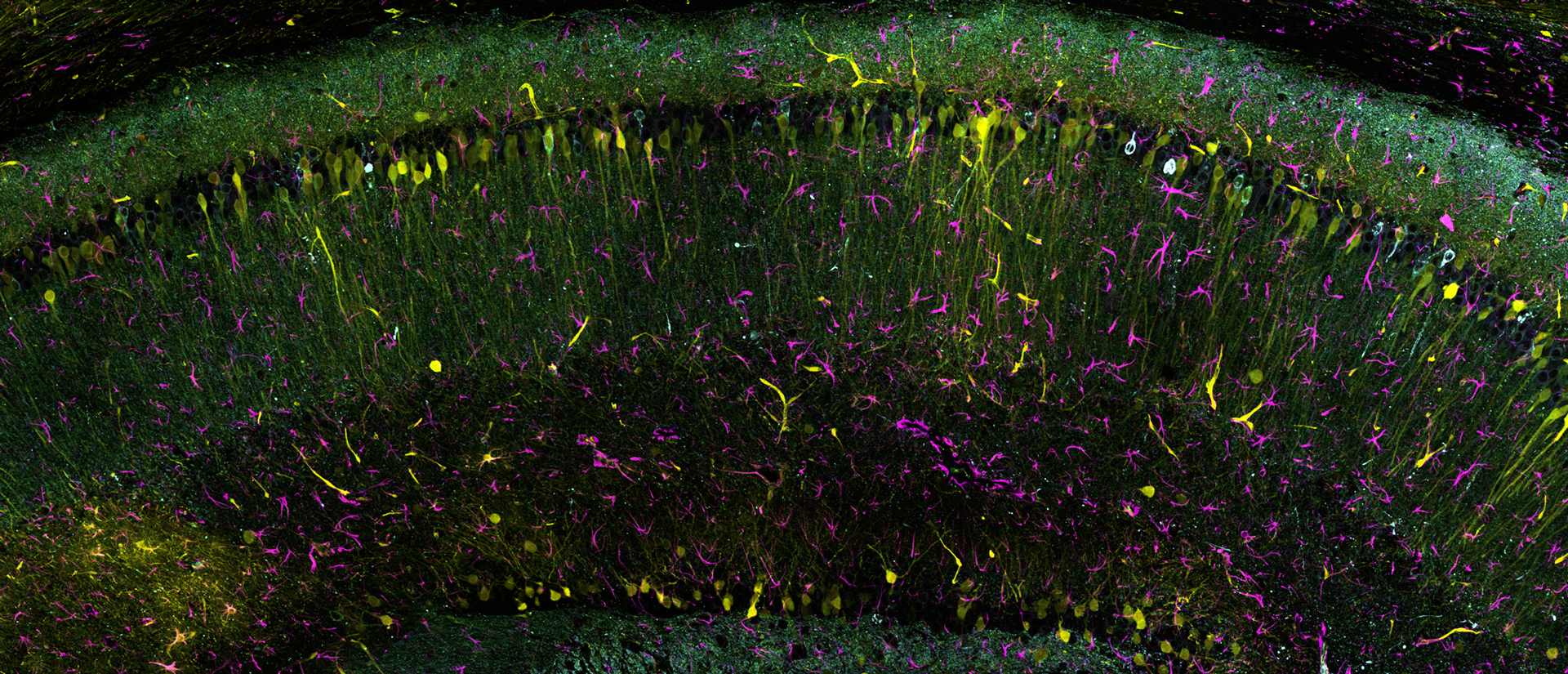
Lateral region of the hippocampus, showing pyramidal cells in yellow and protoplasmic astrocytes in magenta. ©Gabe Luna
Training teachers to profile and promote brain development
The global picture, as you might expect, is mixed. However, some countries are doing notably more, including by training teachers to profile the brains of their students in order to support their development. There is now robust evidence pointing to the existence of specific brain networks responsible for basic capacities for learning mathematics and reading. These capacities may function as part of the “starter kit” for understanding numbers or written words, arising from the biology we are born with. This finding demonstrates the type of “usable knowledge” offered by neuroscience that has important implications for education. It supports the strategy of capitalizing on a student’s neurocognitive capacities whenever possible during instruction. Additionally, it suggests that teachers may be able to detect individual differences in how these capacities are developing in their students, if they are provided with the tools and training required. Teachers are among the best “cognitive enhancers” in the world, helping to change their students’ brains to acquire literacy, numeracy, reasoning skills and a complex range of further competences. Aligned with this, appropriate school-based interventions focusing on a neurocognitive approach are showing promise for enhancing the learning potential of children, including those who develop atypically.
A school-based model for promoting neurocognitive development requires instruments and sustainable designs that fit global and local contexts. Researchers from the educational neuroscience lab at the Cuban Centre for Neuroscience have developed tools for profiling the neurocognitive status of children and for identifying early signs of atypical neurocognitive development. Such a neurocognitive profile facilitates intervention that focuses on individual differences in classroom learning contexts.
The model is supported by current technological advances. Tools for detecting “red flags” are based on mobile solutions, whereas tools for obtaining neurocognitive profiles are computerized tests that facilitate precision and accuracy in the assessment of neurocognitive processes. Both have been developed as client-server applications. Teachers are introduced to the main concepts about the brain and learning through an e-learning platform, using a theoretically guided video game in which they respond to cues for attending to individual differences in the classroom.
The advantages of integrating cognitive neuroscience knowledge with technology can also be seen in the development of new ways of remediating a broad range of developmental disorders. Claims about the general effectiveness of commercial programmes must be carefully scrutinised, but neuroimaging can help provide insight in cases where successful results are reported. For example, a study using an educational computer game based on neuroscientific understanding demonstrated the effects of remediation of problems with dyscalculia in terms of numerical performance and brain function.
When the cognitive and neural data converges in this way, we can be more confident in the effectiveness of the intervention and its underlying theoretical models. Understanding these underlying theoretical models is important for developing technology-based interventions that are effective in the classroom.
Cognitive-based, digitally mediated interventions
One such intervention, in Uruguay, demonstrates just how important it is to have neuroscience and technology inform educational practice. Despite the optimism associated with technology as a learning tool, attempts to apply new technologies to education have often yielded only modest results. However, massive access to digital technology, the availability of a huge corpus of data and growing understanding of the concepts and theories of cognitive neuroscience are creating a wave of new, and hopefully more successful, technology-based educational interventions. Uruguay’s Plan Ceibal project, a scheme that gives one laptop or tablet to each school child, is an excellent example of this.
In principle, two kinds of interventions have the potential to improve educational outcomes; those designed to modify aspects of everyday life and those designed to be used in classrooms. In a study of the first kind of intervention, involving 700 children, researchers at the Universidad de la República (UDELAR) in Uruguay attempted to improve elementary maths performance by developing children’s approximate number system (ANS). The ANS is the brain system we use to estimate quantity without accessing mathematical symbols. The researchers devised a series of games to measure symbolic and non-symbolic maths abilities and used the game PANAMATH to train the ANS. The digital tablets of Plan Ceibal were used in a semi-supervised setting, within classrooms.
All of the children who took part in the study improved in their math tests after the intervention. The increase in performance was higher in schools with low socio-economic status (SES), thus demonstrating that an important tool for helping to close the digital poverty divide can be technology itself.
Another intervention, conducted by the same lab, focused on the study of the cognitive development of executive function in early childhood, in contexts of social vulnerability. The researchers attempted to improve children’s executive function through the use of Mate Marote (MM), a game developed by a team led by Mariono Sigman. Data showed SES was strongly associated with cognitive development. In particular, the performance of children with the lowest and the highest SES differed significantly in tasks of inhibitory control, cognitive flexibility, planning, fluid intelligence and working memory. After eight weeks of playing MM, children from vulnerable contexts achieved significantly better scores in inhibitory control and cognitive flexibility, and the increases were higher for children of the lowest SES schools.
Gamification may help transfer such an approach to situations where children play independently. The researchers at UDELAR are currently testing a prototype app co-created with professional game designers in order to train cognitive control, with the requirement that the game should be engaging for children. The results so far are highly encouraging.
The other type of intervention is to create activities that can be useful in a classroom setting. In line with this, the UDELAR researchers are collaborating with others to create a teacher-friendly platform, using robots to teach programming and so-called “computational thought” to pre-schoolers. They are also exploring the potential of technology to help teach functional programming to high school students and investigating whether this transfers to mathematics. These projects are not merely a new, fashionable techno-optimistic wave, but a genuinely motivated effort to bring what we know about cognitive neuroscience to bear in changing the core and periphery of educational practices.
What is particularly noteworthy about the interventions in Uruguay is that they are selecting and using technologies in a way that is informed by studies that have measured brain activity. In the future, it may be possible for educational technology to interface with the brain more directly. Such “neurotechnology” is becoming cheaper and more portable. It is already possible to see how portable methods of studying brain activity may become part of classroom practice. Studies have shown, for example, that monitoring your own brain activity can help you achieve mental states that are more conducive to creative performance. Adults and school children have demonstrated improved musical performance after training using portable EEG equipment, and similar improvements in creativity have also been found among dancers. In the next few years, we can expect to see devices for measuring brain activity being used more in classroom research.
Measuring arousal to detect “connectedness”
Some simple methods of measuring neurophysiological arousal in the classroom are already providing insights into behaviours that are important for learning. The classroom is a dynamic and highly social environment that features interpersonal interactions between students, as well as between teachers and students. Neuroscience and education researchers are increasingly interested in unobtrusive biometric measures of student engagement in learning, and measures of social interaction or social synchrony in the classroom represent an important factor that underlies successful co-operative learning skills and engagement in group learning activities.
Perhaps the best example of an unobtrusive recording device is the biometric wristband. These lightweight wristbands measure physical activity and the physiological responses of heart rate and sweating (also known as electrodermal activity) which change in response to various psychological and social processes, including arousal, emotional states and attention. A person’s physiological arousal level is measurable by changes in their heart rate and perspiration as they transition between arousal states on a spectrum of disengaged, bored or fatigued at the lower end, to engaged and alert in the middle, through to anxious or stressed at the upper end. Dynamic changes in students’ arousal states, as they increase or decrease in physiological arousal in response to events or activities in the classroom, may represent changes in cognitive engagement or emotional state and can be assessed within particular types of pedagogical practice, or with particular student–student or student–teacher interactions.
A major challenge with biometric measurement is to devise computational methods that can answer research questions of interest to educators, or provide simple metrics that are meaningful and useful in relation to classroom learning or pedagogical practice. One very promising avenue, being explored in Australia, is to examine “connectivity” or synchrony between students through their physiological arousal levels. Such analyses examine the correlated changes in physiological arousal states between students during classroom learning, as biometrics such as heart rate or electrodermal activity appear to simultaneously change between people involved in cooperative work or positive social interaction. The Australian study found that synchronous fluctuations in physiology between students are typically greater when they are engaged in a common task or have a shared focus, such as the teacher during teacher-led instruction. This is a potentially powerful technique to quantify the extent to which students are “connected” with each other and their teacher, or with the ongoing events in the classroom environment.
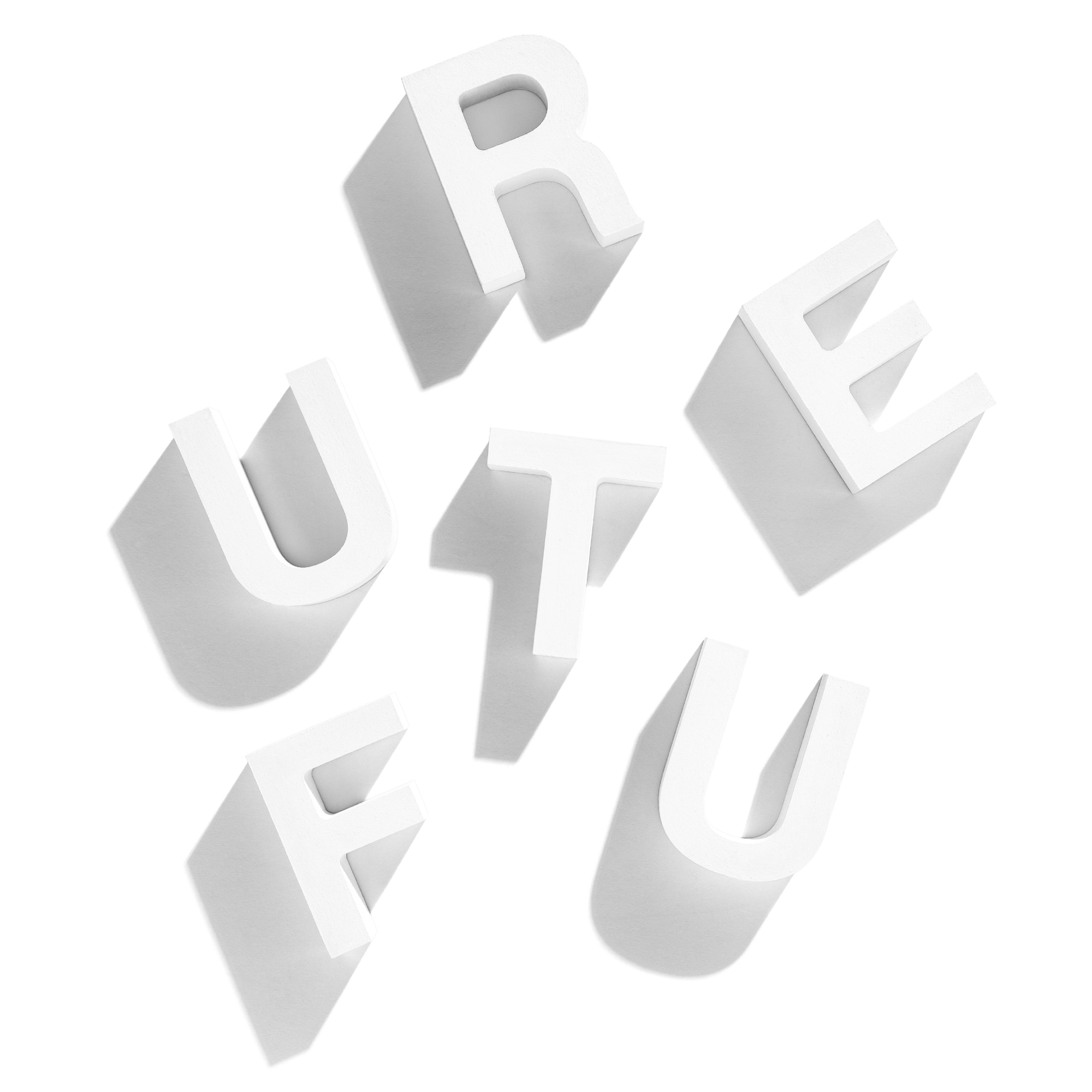
Technology is rapidly advancing, making the idea of personalized neuroscience-informed learning a realizable ambition for the future. ©multipleStudio
The future of neuroscience and technology in education
These four examples demonstrate the huge potential contribution that the convergence of neuroscience and emerging technologies can make to education, through interventions based both in the classroom and in the wider life of the students. Already, it is making a significant difference to learners’ classroom performance and outcomes, as well as to their overall educational prospects, particularly for those from poor or vulnerable backgrounds. It is important to build on this good practice, in ways that are both informed and flexible. Technology is opening up new, often unanticipated, possibilities all the time.
Our accumulating knowledge of the genetic, brain-based, psychological and environmental factors that predict learning may, one day, allow education to be tailored precisely to an individual’s needs. The technology for this important part of the “big data” revolution is not trivial, requiring improved portable neuroimaging for collecting brain data (e.g., neuroheadsets for EEG monitoring) and real-time processing of the data it produces. Gathering and interpreting an individual’s data may also require some further advances in genetic testing technologies. In all of these areas, however, technology is rapidly advancing, making the idea of personalized neuroscience-informed learning a realizable ambition for the future.
Combined with our understanding of cognitive neuroscience, technology is providing new ways to study learning in the brain, helping us identify those in need of extra help and supporting the development of new, scientifically informed technology in the classroom. Through the rolling programme of IBE-IBRO Science of Learning Fellowships, the IBE is looking forward to fully exploiting the opportunities and insights emerging from the fusion of these two exciting fields: education and neuroscience.